By Michael Blanding
In early May of last year, soon after the United States reported its 1 millionth case of COVID-19 nationwide, Dr. Barney Graham, PhD’91, picked up his ringing phone with equal parts hope and trepidation. As deputy director of the National Institutes of Health’s Vaccine Research Center, Graham knew the call could mean one of two things: a breakthrough that might spare the lives of millions around the globe, or a discouraging setback that would allow the accelerating pandemic to continue unchecked for the foreseeable future.
Two months earlier, Graham had overseen the injection of the first volunteers with an experimental vaccine, developed by biotech company Moderna, to combat the novel coronavirus. The patients’ blood serum had been extracted and flown across the country to Nashville, where it was analyzed in the lab of Dr. Mark Denison, director of the Division of Pediatric Infectious Diseases at Vanderbilt University Medical Center.
Now, Denison and Dr. James Chappell, PhD’97, MD’01, research associate professor of pediatrics, were on the phone to Graham to fill him in on the very first results. “Barney,” Denison said, “it looks like it’s working.” Graham let out a breath he felt like he’d been holding for months—ever since SARS-CoV-2 began wreaking havoc on the country’s health. As he examined the images Denison sent, it was clear that the vaccine wasn’t just working—it was blowing the virus away.
To measure vaccine efficacy, scientists create graphs showing how many virus particles the serum kills in various concentrations. The steeper the curve, the more effective the antibodies. “The slope of the curve was very steep,” Graham says. “It not only exceeded my expectations, it exceeded my hopes.”
Recent scientific advances enabled the Moderna vaccine to be developed in a matter of weeks—as opposed to the years typically required for more traditional vaccines—but this was by no means an overnight success story. The key to unlocking a vaccine for COVID-19 was more than 30 years in the making and based on research into a number of other infectious diseases.
In fact, the Moderna vaccine and a similar vaccine developed by Pfizer, as well as an antibody treatment developed by AstraZeneca and the antiviral drug remdesivir, all have their roots in research conducted by Graham and others at Vanderbilt in the 1990s, when no one spared much money—or thought—for coronaviruses. In the ensuing decades, the passion of many of those researchers came together to create treatments for the COVID-19 pandemic in record time.
FROM HIV TO RSV
The story of the development of the coronavirus vaccines started in 1985, when, as chief resident at Nashville General Hospital, Graham walked into the lab of infectious disease researcher Dr. Peter Wright, who served as the first head of the Division of Pediatric Infectious Diseases at Vanderbilt. Graham had grown up a Kansas farm boy with a curious mind.
“On a farm, you spend about half the day fixing equipment in order to get the work done on the back end of the day,” he says over video chat from his book-lined office in Washington, D.C. “I got some really great experiences in figuring out how to solve problems.”
Initially a math major at Rice University in Houston, Graham ended up studying biology and attending medical school at the University of Kansas, where he spent a year working at a virology lab and first became fascinated with infectious diseases.
At Kansas, Graham also met his future wife, psychiatrist Dr. Cynthia Turner-Graham, who suggested in 1979 they try Nashville to obtain positions outside the residency matching program. At the time, couples matching was complicated, but they both ended up in residencies at Vanderbilt—he in medicine and she, later, in psychiatry.
Soon thereafter, Graham began seeing the first ravages of a deadly new disease for which there was no cure. “HIV changed all the paradigms about how you thought about disease,” he says.
Previously, doctors assumed that a patient’s symptoms could be tied back to a single source. With HIV, however, patients were coming in with multiple opportunistic infections—including atypical mycobacteria, pneumocystis pneumonia and a range of other illnesses—as a result of their compromised immune systems. It was years before they would fully understand the concept of acquired immune-deficiency syndrome (AIDS) and its root cause, human immunodeficiency virus.
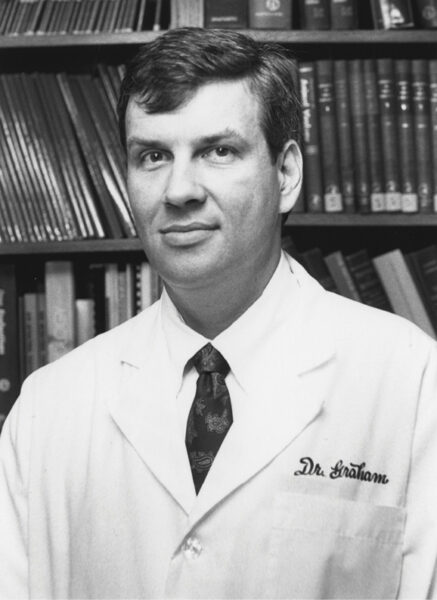
Graham asked for a position in Wright’s lab for the research phase of his infectious diseases fellowship. Instead of HIV, however, Wright asked for help with another infectious disease: respiratory syncytial virus, or RSV, a common respiratory virus that could be fatal in children. Tragically, a vaccine that had been developed for the virus in the 1960s caused the disease to worsen in some children, leading to several deaths. Graham became obsessed with understanding why and conducted basic immunology research to better understand how the virus operates.
When a virus enters the body, it attaches to cells with a protein and then transforms itself to release its deadly viral payload. “These proteins are designed like little motors,” Graham says. “They reach out and grab the cell, pull the cell and virus membranes together, and allow fusion to occur.”
The problem is, killing a virus with heat to make a more traditional vaccine transforms it into its “post-fusion” form—that is, what it looks like after it attacks a cell. In the case of RSV, the protein goes from looking like a broccoli floret to more like a golf tee. Those shapes are important, because when our immune systems recognize the shape and surface contours of the protein as foreign, they react by producing antibodies that attach to the shape.
When injected into the body, the more traditional RSV vaccine caused the formation of antibodies that were totally ineffective in attacking the pre-fusion virus—and may even have done damage to children by flooding the lungs with nonfunctional antibodies. While others continued trying to make vaccines for the post-fusion form, Graham became determined to create a vaccine for the pre-fusion form. He started studying the cellular structure of the virus and conducting experiments in mice.
In 1986, when HIV research was drying up funding for other diseases, Graham took the unusual step of pursuing a Ph.D. in microbiology and immunology. At the same time, he accepted a faculty position at Vanderbilt’s School of Medicine with the support of Dr. John Oates, who was then chair of the Department of Medicine.
Meanwhile, the AIDS epidemic was spiraling out of control, and Wright asked Graham to help with clinical trials for potential vaccines. Graham split his time between conducting basic research on RSV and running vaccine trials for HIV.
“Most people, if they focus in one area, have trouble keeping up with another,” says Dr. Kathy Neuzil, MPH’98, the first research fellow in his RSV lab. “But he was a really good immunologist, and a really good virologist, and a really good clinical doctor. There are few people who can do that.”
Though he was driven, she also found him mild-mannered and amiable. “If you find someone who doesn’t get along with Barney, let me know,” says Neuzil, the Myron M. Levine, MD, DTPH Professor in Vaccinology and director of the Center for Vaccine Development and Global Health at the University of Maryland.
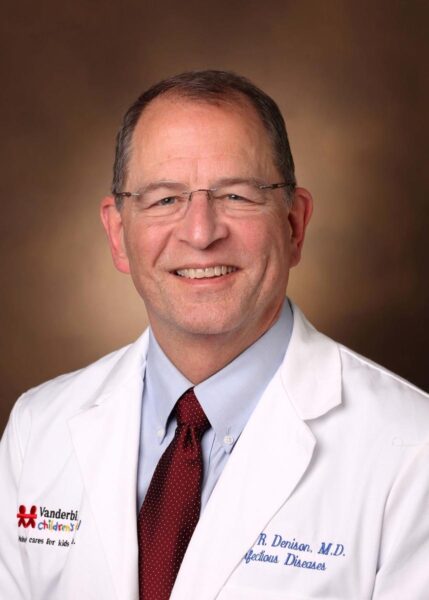
“Barney represented to me the ultimate synthesis of a visionary, brilliant scientist, who is also exceptionally kind, generous and collaborative,” says Denison, another Kansas University grad who arrived at Vanderbilt in 1991 and lived across the street from Graham. “I don’t remember him ever putting down the science of anybody.”
Denison, who now holds the Edward Claiborne Stahlman Chair in Pediatric Physiology and Cell Metabolism and is professor of pathology, microbiology and immunology at Vanderbilt, knows something about pursuing unpopular viruses. He became fascinated with the complex genetic material of coronaviruses—pathogens that cause extreme infections in animals but often only mild colds in humans.
“I’ve heard it a million times—who really cares about coronavirus?” he says. “It was very hard to get the research into high-impact journals because it was not sexy.”
CORONAVIRUS BREAKTHROUGH
Wright, Graham, Neuzil and Denison formed the nucleus of a unique community, which also included Dr. Kathryn Edwards and Dr. Bill Gruber. Edwards is now the Sarah H. Sell and Cornelius Vanderbilt Professor of Pediatrics at the School of Medicine. Gruber, who had been Graham’s roommate at Rice and had come to Vanderbilt to work on vaccine trials for several viral diseases affecting children, is now the senior vice president of vaccine clinical research and development at Pfizer.
The group was unusually collaborative—Graham and Neuzil were adult physicians working on a childhood disease, while Wright, Edwards and Gruber were pediatricians running trials on an adult vaccine. Graham became the bridge among the various disciplines of adult and childhood diseases, as well as the basic science and clinical research.
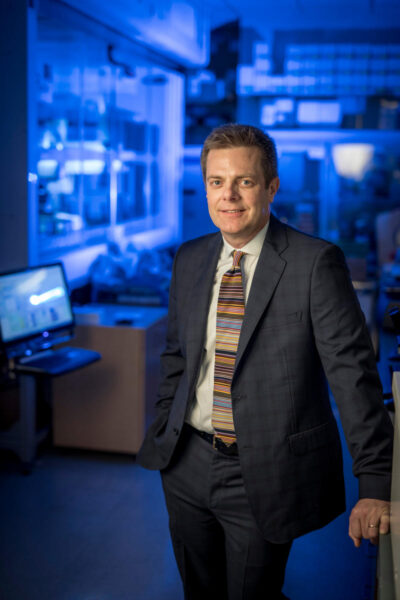
“He was doing RSV basic science and HIV clinical vaccine development, and so they were different targets and almost different disciplines,” says Dr. James Crowe, Ann Scott Carell Chair, professor of pediatrics and director of the Vanderbilt Vaccine Center. “Barney was living in both worlds—he was a liaison, a connector.” In 1995, Graham helped recruit Crowe to Vanderbilt from the National Institutes of Health, where he was researching RSV.
Graham’s work on HIV caught the attention of the National Institute of Allergy and Infectious Diseases, and he left Vanderbilt to join its Vaccine Research Center in 2000. He never abandoned his research on RSV, however.
For years, scientists had tried to engineer a protein for HIV that would create potent neutralizing antibodies in patients. While they hadn’t yet succeeded, Graham began applying those protein engineering techniques to RSV. He and a young postdoc—Jason McLellan, who was working in Peter Kwong’s laboratory at the Vaccine Research Center—focused on the so-called F protein, which sticks out of the RSV and is used to fuse with cells. By capturing the structure of F in its pre-fusion form, they found ways of modifying the protein from the inside to stabilize its shape, creating a surface that would produce functional antibodies.
Graham immediately recognized that this level of precision in protein engineering was a game-changer in developing vaccines for RSV and other enveloped viruses, including coronaviruses. The breakthrough transformed their research, boosting immunity in animals by more than fiftyfold.
By then, coronaviruses were becoming an increasing threat to humans—starting with the SARS outbreak in 2002 and MERS (Middle East Respiratory Syndrome) in 2012, which caused 40 percent fatality in those infected. Coronaviruses get their name from the spike proteins that cover their exterior, making them look like crowns (corona in Latin) and latching onto cells much like the F protein in RSV.
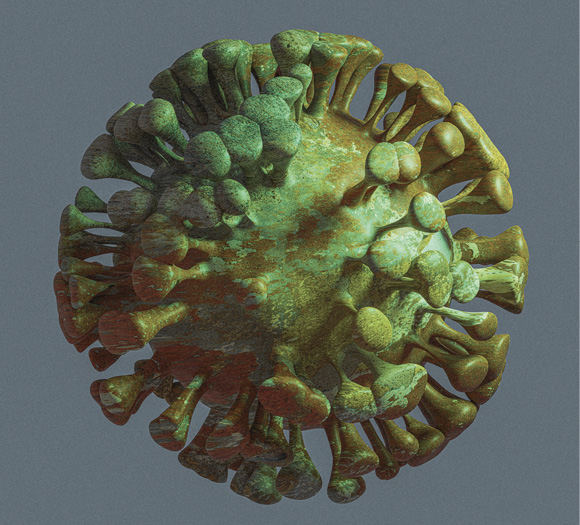
Having little success with SARS and MERS initially, Graham and McLellan turned to the more common coronavirus HKU1, quickly stabilizing the spike protein in its pre-fusion form by adding two molecules of the amino acid proline—the most rigid of the 20 amino acids—to the top of its central helix. With that stabilized spike, they found they could produce 50 times more spike protein and much better neutralizing antibodies.
Despite the radical implications, Graham and McLellan struggled to get their research published. They were rejected by five scientific journals before they were successful in 2017 with the Proceedings of the National Academy of Sciences. The ominous emergence of new deadly coronaviruses did little to sway the field from downplaying their relevance.
“Everybody said, ‘Why are you working on coronaviruses? They just cause the common cold,’” Graham says. Yet he and McLellan persisted.
Meanwhile, Graham was applying other techniques to combat the mosquito-borne Zika virus. For this vaccine, he and other NIH colleagues worked to rapidly develop a vaccine delivered by DNA. At the same time, they partnered with a new Massachusetts biotech company, Moderna, which had developed a technique to create vaccines quickly. Instead of using DNA to produce virus proteins inside the body, the researchers used messenger RNA to deliver the instructions for cells to make the same proteins. They found the mRNA approach for vaccination to be more potent than the DNA one.
“The cell becomes the manufacturing center,” Graham explains.
By the time the researchers showed success with the new technique in monkeys, the Zika epidemic had waned. But in 2017, having been impressed with mRNA, they worked with Moderna on a plan to combine the precision antigen design with the rapid and potent mRNA delivery. They focused on two virus families: coronaviruses (represented by MERS-CoV) and paramyxoviruses, for which Nipah virus—which had caused periodic outbreaks in Asia—was the prototype.
By early 2020, Graham’s group had designed the vaccine, and Moderna was ready to manufacture the Nipah mRNA and take it into a human clinical trial. But on Jan. 6, 2020, word came that Chinese scientists had isolated the virus that had caused an epidemic in Wuhan and was now in danger of creating a deadly worldwide pandemic.
“I called Jason and said, ‘Get back in the saddle,’” Graham remembers. “‘We’re going to have to solve another structure.’”
A RACE TOWARD VACCINATIONS
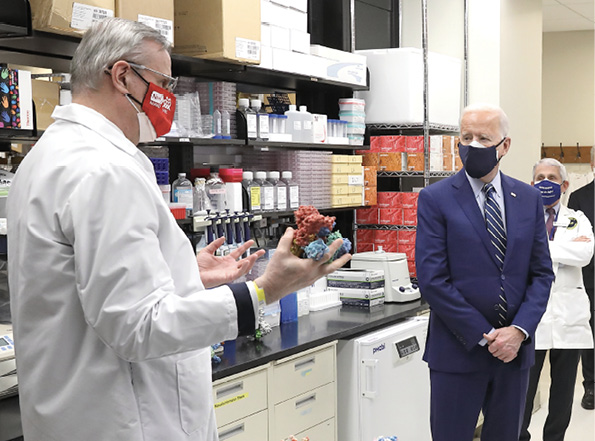
By then, Graham and McLellan had the process down. On Jan. 10, Chinese scientists released the genetic sequence of the new virus, SARS-CoV-2. On the morning of Jan. 11, Graham’s lab and McLellan’s lab, now at University of Texas–Austin, worked together to design sequences to make the protein for solving structures, developing assays and immunizing mice.
This time, Graham vowed, they wouldn’t be late.
While McLellan was revealing the atomic structure of the new spike, Graham’s group provided the protein sequence for Moderna to begin manufacturing mRNA that could be injected into mice, as well as an assay to determine its effectiveness. Within two weeks, they started animal trials—an unheard-of speed for a newly discovered virus. But Graham was confident.
“Even though we didn’t have any antibodies yet,” he says, “you could see the protein was in the right shape, and it was likely going to work.”
By now, the first cases of COVID-19 had emerged in the United States, adding new urgency to their mission. Graham collaborated with another group at NIH from the Division of Microbiology and Infectious Diseases that arranged to test the vaccine in humans just as the first animal data began showing efficacy. Neuzil worked with the team to design a trial of the first eight subjects in Seattle on March 16, followed shortly by another 37.
Usually it takes anywhere from 18 months to three years to develop a vaccine that can be used in people. In this instance, it took just a few weeks.
“Within 41 days after we gave Moderna the protein sequence, they gave us back an mRNA product that could be put into people,” Graham says proudly. “We did animal and human studies in parallel, at the same time, so we would always have the data we needed to start the next stage.”
It was the serum from those first eight subjects that Mark Denison’s lab analyzed two months later, delivering the good news to Graham that the vaccine was effective. “We were the very first humans to see the effect of this vaccine actually killing COVID,” Denison says. “It was a very emotional moment.”
Denison himself hadn’t been idle in tackling coronaviruses in the intervening years. Ever since the emergence of SARS in 2002, he had been warning about the possibility of a global coronavirus pandemic. “It’s like saying a Category 5 hurricane is going to hit New Orleans or Miami,” Denison says. “You know it’s going to happen, and you should be
prepared for it now.”
In recent years, Denison had been testing compounds to treat illnesses caused by coronaviruses. One promising treatment was called nucleoside analogues—compounds that mimic the genetic code of a virus, infiltrate it and kill it. Coronaviruses, however, have a unique ability to “proofread” their genes and remove nucleotides that don’t belong.
In 2014, Denison’s team tested a new compound by Gilead Sciences, now called remdesivir, and showed it was the first known drug to bypass coronavirus proofreading and thus stop the virus from replicating. Between 2014 and January 2020, the Denison lab team and their collaborators at University of North Carolina showed that remdesivir killed every known coronavirus, and it prevented and treated SARS and MERS in animals. This amazing timing led to clinical trials against COVID-19 in China and the United States by February 2020. By May, the Food and Drug Administration had issued an emergency authorization to use remdesivir to treat the virus in humans, followed by its licensure as the first COVID-19 antiviral.
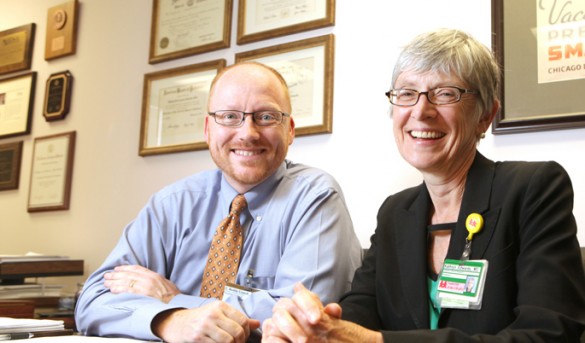
With Graham’s invitation and support, Denison became involved in the design of clinical trials for the Moderna vaccine, and James Chappell led testing in the Denison lab of the phase 1 vaccine volunteers’ serum, from 1,000 patients across the country. Dr. Buddy Creech, BS’95, MPH’06, associate professor of pediatrics and director of the Vanderbilt Vaccine Research Program, directed phase 3 trials of the Moderna vaccine, and Kathryn Edwards, the founder and former longtime director of VVRP, served a critical role with data safety and monitoring boards at the national level for safety studies of the vaccine.
Once again, the trial results dramatically exceeded expectations. “I was expecting 70 to 75 percent, hoping for 80 percent, and we got 95 percent,” Graham says.
Meanwhile, Graham’s former roommate Bill Gruber was testing Pfizer’s vaccine, also based on Graham’s techniques of stabilizing the spike protein, while James Crowe was pursuing a completely different tack to combat coronavirus with British pharmaceutical company AstraZeneca. Crowe had become fascinated with the phenomenon of monoclonal antibodies, which are custom-designed outside the body and then injected to attack a virus by inhibiting its ability to grow and reproduce. Theoretically, monoclonal antibodies can be used as a vaccine to create immunity and also as a treatment after a person is exposed or infected.
Last year, Crowe’s lab ran a simulation to show it could create monoclonal antibodies for a new virus in 78 days. When the COVID-19 coronavirus hit in early 2020, it cut that time by two-thirds, synthesizing antibodies in just 24 days. (The treatment is currently in phase 3 trials.) Crowe doesn’t think it’s an accident that the legacy of Vanderbilt’s unique vaccine program in the 1990s led to such quick responses—with vaccines, antivirals and antibodies—decades later.
“People say how could they make vaccines for corona so fast,” he says. “Well, they did it based on more than 30 years of research on RSV, HIV and MERS.”
Denison marvels that in the months prior to the COVID-19 outbreak, all of the researchers had made major breakthroughs that allowed them to accelerate a response. “All of those years where you labor on this fundamental biology and genetics of viruses, trying to get support for this work, or trying to convince people to let you test their compounds,” he says, “and then all of these papers were published in the months before.”
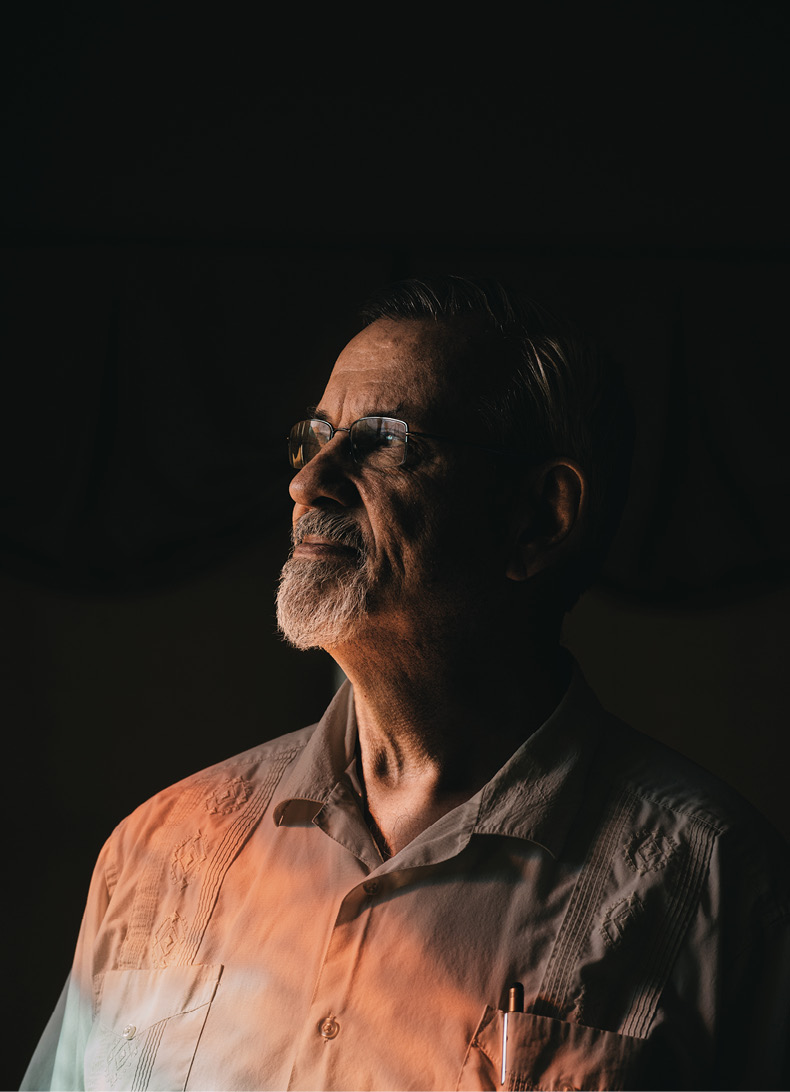
says Graham, who advocates for more basic research into all infectious diseases. (Greg Kahn)
Neuzil spent 2020 overseeing clinical trials, thinking the best-case scenario was to see vaccine approval by the end of the year. On Dec. 31, she got her first injection and sent a photo to Gruber and to Graham, who wrote back right away: “Seeing pictures of people being vaccinated is becoming one of my favorite pastimes.”
As pleased as Graham, who received the 2021 Vanderbilt Distinguished Alumnus Award, has been to see his labors bear fruit in potentially saving thousands—if not millions—of lives, he also sees the story of the coronavirus vaccine development as a cautionary tale.
“As bad as this is, we were lucky this was a coronavirus,” he says, noting that there are 26 families of viruses that infect humans, many of which are still mysterious to scientists. “If it was a bunyavirus or an arenavirus, we would have been lost for months or a year or two just trying to get the right thing made.”
In that sense, the COVID-19 pandemic was a wake-up call, signaling the need for more basic research into all infectious diseases, not just the ones that are trendy. That’s the only way, he says, that when the next worldwide outbreak occurs, we’ll be ready to act.
Michael Blanding is an award-winning Boston-based journalist whose work has appeared in The New York Times, Wired, Slate, The Nation, and The Boston Globe Magazine. Currently, he is a senior fellow at the Schuster Institute for Investigative Journalism at Brandeis University.
Watch a Vanderbilt Chancellor’s Lecture Series discussion featuring Graham, Neuzil and Denison: