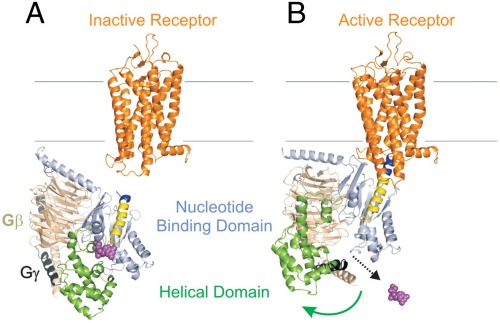
Watch an animated version of this event created by Associate Professor of Chemistry Jens Meiler and his colleagues. (Requires QuickTime)
To smell or see or taste, to respond to danger with a racing heart, to focus the mind or experience joy — all require marvelous intracellular switches known as G proteins.
Although the structure of G proteins was solved nearly 20 years ago, exactly how they are “turned on” by their G protein-coupled receptors (GPCRs) has remained a mystery — until now.
Reporting in the Proceedings of the National Academy of Sciences earlier this year, researchers at Vanderbilt University and the University of California, Los Angeles describe how a G protein “swings open” after binding to its GPCR.
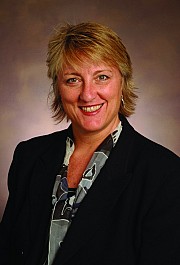
This is a fundamental discovery, said Vanderbilt Pharmacology Chair Heidi Hamm, who led the research with Wayne Hubbell, Jules Stein Professor of Ophthalmology and Distinguished Professor of Chemistry and Biochemistry at UCLA, and Jens Meiler, associate professor of chemistry at Vanderbilt.
It reveals a mechanism that may be essential for every G protein-activated pathway, Hamm said, and suggests that G proteins could be targets for new, more effective drugs.
Hormones, neurotransmitters and more than half of all drugs on the market accomplish their physiological feats by binding to and activating GPCRs, which loop through both sides of the cell membrane.
Once the signal is received, the receptor changes shape, enabling it to bind — and activate — G proteins inside the cell. That “throws the switch,” and unleashes a cascade of downstream events ending up with a fluttering heart or sweet-smelling sensation.
Because GPCRs activate multiple G protein-signaling pathways, drugs that target these membrane-
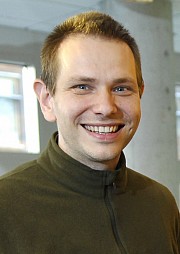
embedded receptors are a little like using a shotgun when a rifle would do. Tackling G protein-activated pathways inside the cell could lead to new classes of laser-like drugs with fewer side effects.
G proteins consist of three subunits, alpha, beta and gamma, and are named for the energy-transferring molecules they carry — guanosine diphosphate (GDP) and guanosine triphosphate (GTP).
In its inactive state, the G protein carries GDP. But when activated by its receptor, the “Galpha” subunit jettisons its low-energy GDP cargo in favor of a higher-energy molecule of GTP, and splits off from the beta/gamma subunits.
In 1993, while at the University of Illinois at Chicago, Hamm solved the structure of the Galpha subunit with the late Paul Sigler and his colleagues at Yale.
At about the same time, Hubbell and his colleagues at UCLA were developing a powerful new technique, called site-directed spin labeling or SDSL, to study how protein structure changes over time.
Not long after Hamm arrived at Vanderbilt in 2000 to chair the Department of Pharmacology, she and Hubbell and their colleagues began working together to solve a biological mystery.
They knew that when a G protein called transducin binds to and is activated by its light-sensitive receptor — rhodopsin — in rod cells in the retina, it triggers a cascade of events that enables vision in dim light or at night. But how exactly does activation happen?
In 2006, using SDSL along with double electron-electron resonance (DEER) spectroscopy, the researchers pinpointed the site of the activation to a critical region of the Galpha subunit.
In the latest study, they combined DEER, biochemical analysis and state-of-the-art molecular modeling to measure the distance between two “domains,” or chains of amino acids, of the Galpha subunit — before and after activation.
They discovered that the domains swing widely apart upon activation, allowing release of the GDP molecule — a crucial step in the transmission of the visual signal.
“Not only is the structural change large, but apparently there is a high degree of molecular flexibility in the complex. This is unexpected based on conventional thinking in protein science, and is something that cannot be directly observed in crystal structures. It is very likely that such flexibility will turn out to be important for function,” said Hubbell, the Jules Stein Professor of Ophthalmology and Distinguished Professor Chemistry and Biochemistry at UCLA.
To confirm the importance of the conformational change, Hamm and her colleagues showed that they could block G protein activation by inserting chemical cross-links or “straps” that prevented the domains from separating.
Their finding was reinforced by a report published last month in the journal Nature , which demonstrated domain separation in another G protein, Gs, upon binding to its GPCR, the beta-2 adrenergic receptor.
“Back in 1993 when we solved the first crystal structure of (the alpha subunit), we predicted that the two domains must open to allow GTP-GDP exchange,” said Hamm, the Earl W. Sutherland Jr. Professor of Pharmacology. “It is gratifying to actually be able to demonstrate this domain movement biochemically.”
Anita M. Preininger, research assistant professor of pharmacology at Vanderbilt, Vanderbilt graduate student Nathan Alexander, and Ned Van Eps of the UCLA Jules Stein Eye Institute were joint first authors of the PNAS paper. Vanderbilt’s Ali I. Kaya, and Scott Meier also contributed.
The research was supported by the National Institutes of Health.