UPTON, NY — Scientists from Vanderbilt University and the University of Colorado have discovered that surprisingly small droplets of a nearly perfect fluid appear in nuclear collisions recorded by the PHENIX detector. Tiny projectiles seem to be creating a quark-gluon plasma, a primordial cosmic soup from which all the visible matter and energy in the universe originally formed.
Their work, outlined in the paper “Creation of quark-gluon plasma droplets with three distinct geometries,” was published today in the journal Nature Physics.
Scientists had been puzzling over unexpected observations that some believed were tiny droplets of subatomic particles behaving like nearly perfect fluids, while others thought droplets that small could not exist. This led Vanderbilt Professor of Physics Julia Velkovska at Vanderbilt University and Professor Jamie Nagle at the University of Colorado, Boulder, to search for proof that a quark-gluon plasma was in fact being created.
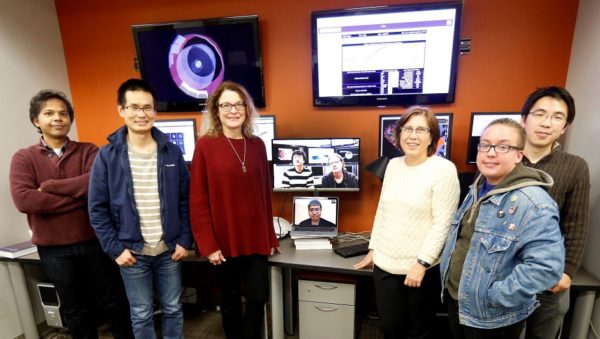
The two research groups analyzed data collected by the PHENIX collaboration, an international team of nuclear physicists who study matter at extreme conditions at the RHIC particle accelerator at Brookhaven National Laboratory. The team studied single protons, two-particle deuterons and three-particle helium-3 nuclei colliding at nearly the speed of light with large gold nuclei.
Particles flying outward from these collisions provided evidence about the conditions inside the submicroscopic fireballs within the collisions. The team led by Velkovska and Nagle discovered that these escaping particles emerged in distinctive patterns that are produced by quark-gluon plasmas. Measurements at RHIC show that in collisions between large nuclei, quark-gluon plasma can form and it flows like a liquid. However, many scientists doubted that this could happen in small collisions.
Velkovska, deputy spokesperson for the RHIC PHENIX collaboration, said the team compared measurements of two different types of particle flow (elliptical and triangular) from all three collision systems with predictions based on the initial geometry.
“The latest data — the triangular flow measurements for proton-gold and deuteron-gold collisions newly presented in this paper — complete the picture,” Velkovska said. “We have analyzed two flow patterns in three different systems and in all six cases, the measurements match the predictions based on hydrodynamics, the theory of fluid flow. We are seeing very strong correlations between initial geometry and final flow patterns, and the best way to explain that is that quark-gluon plasma was created in these small collision systems. This is very compelling evidence.”
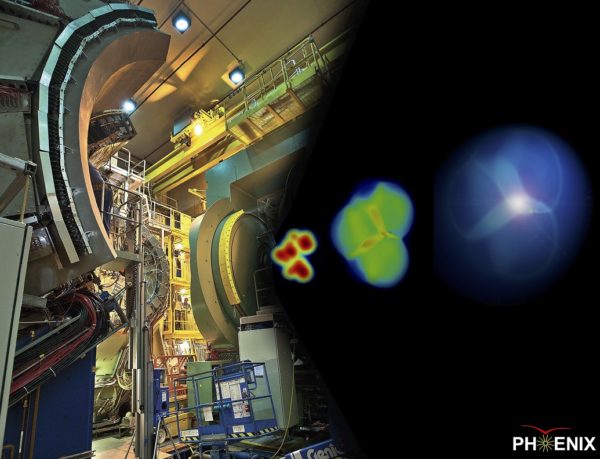
The analysis of the flow patterns in proton-gold collisions was the subject of the 2018 Vanderbilt doctoral thesis of Qiao Xu, who was co-advised by Velkovska and Stevenson Professor of Physics Victoria Greene. The combined analysis of all three collision systems and the detailed comparison to theoretical models, including some that do not rely on quark-gluon plasma formation to create the flow patterns, is the subject of the doctoral thesis of Sylvia Morrow, who co-chaired the paper preparation group within PHENIX.
Morrow’s analysis concluded that theories based on quantum interactions among the gluons building up the initial projectile and target nuclei give a poor description of the data, while hydrodynamical models which include the formation of a quark-gluon plasma droplet match-up very well.
Greene and Velkovska worked on the 2005 PHENIX team that discovered that the quark-gluon plasma is the most perfect liquid known in nature. This state of matter is now a well-established phenomenon in collisions between two gold nuclei, where the intense energy of hundreds of colliding protons and neutrons melts the boundaries of these individual particles and allows their constituent quarks and gluons to mingle and interact freely.
According to Greene, the ongoing work has major implications for the field.
“How small can a system be and still exhibit collective behavior? The idea that you can replicate the Big Bang in miniature in the laboratory and understand that the early state of the universe was this perfect liquid state could inform cosmological models,” she said.
The Vanderbilt team is now tooling up to build particle detectors for a new experiment, sPHENIX, which will have state-of-the-art detectors and superb data rate capabilities, which will allow the researchers to continue their exploration of emergent phenomena in high energy nuclear physics.
This work was supported by the Department of Energy Office of Science, and by all the agencies and organizations supporting research at PHENIX.
Karen McNulty Walsh of Brookhaven National Laboratory contributed.